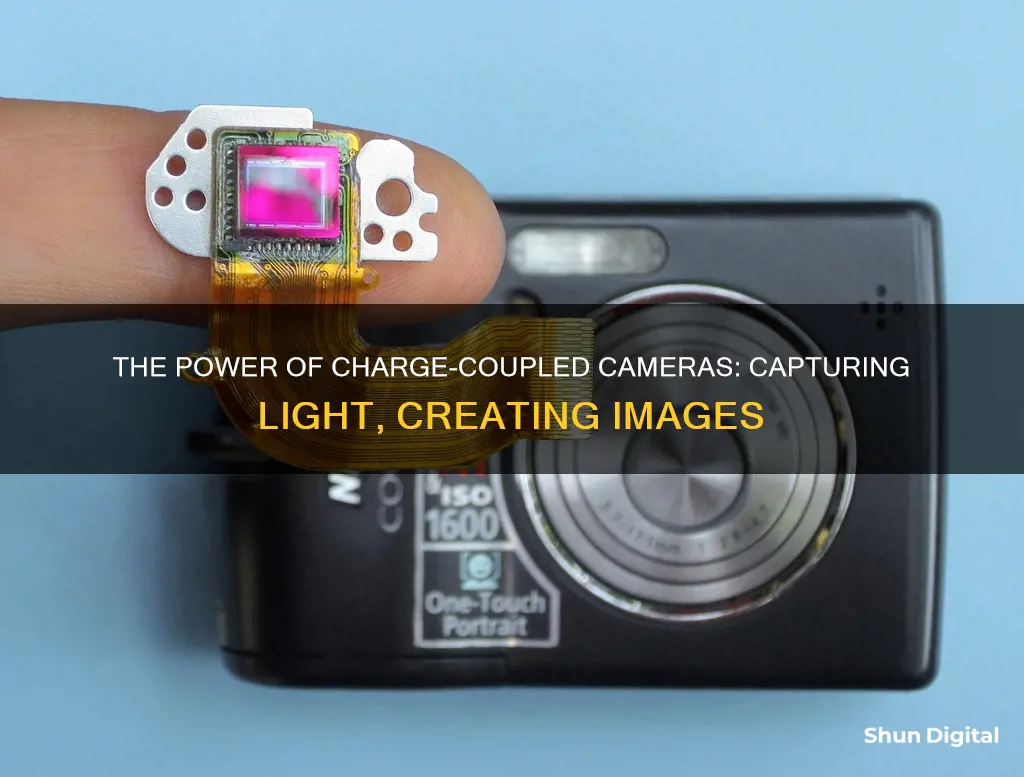
Charge-coupled devices (CCDs) are light-sensitive integrated circuits that capture images by converting photons to electrons. They are commonly used in digital cameras and other devices such as scanners, bar code readers, microscopes, medical equipment, and telescopes. CCDs were the sensors of choice for many years due to their ability to deliver high-quality, low-noise images. However, they are now being steadily replaced by complementary metal-oxide-semiconductor (CMOS) technology, which is cheaper, easier to manufacture, and more energy-efficient. Despite this, CCDs still offer superior precision and sensitivity, making them the preferred choice for applications that demand high image quality, such as in medical, scientific, and industrial equipment.
Characteristics | Values |
---|---|
Definition | A charge-coupled device (CCD) is a light-sensitive integrated circuit that captures images by converting photons to electrons. |
Invention | Invented in 1969 at Bell Labs by George Smith and Willard Boyle. |
Use Cases | Used in digital cameras, scanners, bar code readers, microscopes, medical equipment, and astronomical telescopes. |
Image Quality | Creates high-quality, low-noise images. |
Resolution | Capable of producing images with over one million pixels. |
Power Consumption | Uses a process that consumes a lot of power, up to 100 times more than CMOS sensors. |
Manufacturing | More expensive and challenging to manufacture compared to CMOS sensors. |
Heat Generation | Generates more heat compared to CMOS sensors. |
Sensitivity | High sensitivity to light, with pixels collecting photons and generating electrons. |
Precision | Offers high precision and accuracy, making it suitable for applications that demand exactness. |
Dynamic Range | Has a wide dynamic range, capturing both bright and dark areas in an image effectively. |
Colour Reproduction | Able to reproduce colours accurately by using a Bayer mosaic filter or other colour filtering techniques. |
High-quality image data
Charge-coupled devices (CCDs) are light-sensitive integrated circuits that capture images by converting photons to electrons. They are widely used in applications where high-quality image data is required, such as in professional, medical, and scientific fields. CCDs are composed of an array of linked capacitors, with each capacitor transferring its electric charge to a neighbouring one. This process is controlled by an external circuit, allowing the charges to be read out and converted into a digital copy of the light patterns.
CCDs offer several advantages for high-quality image data. Firstly, they have extraordinary dynamic range, spatial resolution, spectral bandwidth, and acquisition speed. This enables them to capture fine specimen details and provide excellent resolution. The light sensitivity and collection efficiency of CCDs are also impressive, with some systems having a film speed rating equivalent to ISO 100,000. This high sensitivity allows CCDs to respond to near-infrared light, making them suitable for infrared photography and night-vision devices.
Another advantage of CCDs is their ability to maintain linearity over a wide range of light intensities. This linear response contributes to the superior performance of CCDs and enables their use as imaging spectrophotometers. Additionally, CCDs have a high quantum efficiency, which is a measure of the likelihood that a photon will be captured and converted into charge carriers. While most CCDs have quantum efficiencies of 40-50%, newer designs can reach up to 80%.
The full-well capacity (FWC) of CCDs is another factor that contributes to high-quality image data. The FWC represents the maximum number of electrons a pixel can hold, and it affects the dynamic range and signal-to-noise ratio of the image. CCDs with larger pixels or specialised designs can have higher FWC values, improving their performance in low-light conditions.
CCDs also benefit from cooling, which reduces the array's dark current and improves sensitivity to low light intensities. This is particularly useful for ultraviolet and visible wavelength applications. By cooling the CCD, the dark noise is minimised, resulting in a negligible impact on the image quality.
In summary, CCDs offer high dynamic range, excellent resolution, high quantum efficiency, and improved performance in low-light conditions through features like full-well capacity and cooling. These advantages make CCDs a preferred choice for applications requiring high-quality image data.
Charging Your SimpliSafe Outdoor Camera: A Quick Guide
You may want to see also
Low noise
CCDs are highly sensitive photon detectors that can be used to build up an image of the scene of interest. Each CCD is divided into a large number of light-sensitive small areas known as pixels. When a photon of light falls within the area defined by one of the pixels, it is converted into one or more electrons, and the number of electrons collected is directly proportional to the intensity of the scene at each pixel. This results in a high level of detail and dynamic range.
CCDs are composed of a thin silicon wafer that captures light by allowing photons to bounce off its surface, releasing electrons in the process. These electrons are then collected and stored in tiny positively charged capacitors known as photosites. The use of a single amplifier circuit to amplify signals, as opposed to the multiple local amplifiers in CMOS sensors, contributes to the low noise levels in CCDs.
CCDs are also cooled to reduce dark noise, which is caused by the thermal generation of electrons and holes in the silicon substrate. This improves the charge transfer efficiency, resulting in minimal charge loss during the thousands of transfers required for megapixel sensors. Additionally, the application of microlenses on the CCD surface improves light collection efficiency, further enhancing the low-noise performance of CCDs.
The high quantum efficiency of CCDs, which can reach over 80%, also contributes to their low-noise characteristics. This efficiency is further improved by the use of materials such as indium-tin oxide in the gate structures, which have higher transparency in the blue-green spectral region. Back-thinned CCDs, where most of the silicon substrate is removed by etching, can achieve quantum efficiencies of up to 90%.
Overall, the combination of sensitive photon detection, efficient charge transfer, and high quantum efficiency makes CCDs ideal for applications requiring low-noise imagery, such as microscopy and space photography.
Is Your SQ8 Camera Charged? Check This Way
You may want to see also
Light sensitivity
CCDs have a high light sensitivity and light collection efficiency, with a quantum efficiency of up to 70%. This means they are far more efficient than photographic film, which captures only about 2% of incident light. CCDs can also detect near-infrared light, enabling infrared photography, night-vision, and zero lux video recording.
CCDs are widely used in applications where high-quality image data is required, such as in professional, medical, and scientific fields. They are particularly useful in low-light conditions and have been used in astronomy, medical equipment, and digital cameras.
The light sensitivity of CCDs can be further enhanced by cooling the devices, which reduces thermal noise and improves their sensitivity to low light intensities. Cooling can be achieved using liquid nitrogen or Peltier junctions (thermoelectric coolers).
CCDs have been largely replaced by CMOS sensors in consumer products due to their lower cost and ease of manufacturing. However, CCDs are still favoured in applications requiring high precision and sensitivity, such as in medical and scientific equipment.
London Congestion Charge Cameras: Locations and Fines
You may want to see also
Precision
The precision of charge-coupled devices (CCDs) is closely tied to their ability to capture high-quality images with low noise. This precision is achieved through the following key factors:
Pixel Structure and Size: CCDs are made up of an array of light-sensitive elements called pixels, which are etched onto a silicon surface. The size and structure of these pixels play a crucial role in the precision of the CCD. Smaller pixels allow for higher spatial resolution, capturing finer details in the image. In scientific and industrial applications, larger pixels are often used to maximize dynamic range, sensitivity, and signal-to-noise ratio.
Charge Generation and Conversion: The precision of CCDs relies on their ability to accurately convert photons into electrons. Each pixel collects photons and generates an electric charge proportional to the intensity of light it captures. This charge generation process is crucial for the overall precision of the image.
Charge Transfer and Readout: After charge generation, the charges are transferred to a readout node while maintaining their integrity. The precision of this transfer process is essential to ensure that the charges from each pixel are accurately measured. The charges are then converted into voltage signals, which are digitized and stored as image data.
Noise Reduction: CCDs are known for their low-noise performance compared to other sensor technologies. This precision in noise reduction is achieved through various methods, such as cooling the CCD to reduce dark current and improve sensitivity. Additionally, CCDs with built-in microlenses improve light collection efficiency, resulting in higher signal-to-noise ratios.
Dynamic Range: The dynamic range of a CCD refers to the ratio of its full well capacity (maximum signal intensity) to the read noise (minimum detectable signal). A higher dynamic range indicates a more precise sensor, as it can capture a wider range of light intensities. This dynamic range is crucial for applications that require accurate quantitative measurements.
Applications: The precision of CCDs makes them suitable for a wide range of applications, including medical imaging, scientific research, industrial equipment, and astronomy. Their high sensitivity and low-noise characteristics allow them to capture fine details and quantitative data in various fields.
Smart Guide: Universal Charger for Fuji NP-W126
You may want to see also
High dynamic range
CCDs have a high dynamic range, which is a measure of the maximum signal intensity variation that can be quantified by the sensor. The dynamic range is specified by the ratio of pixel full well capacity (FWC) to the read noise. This value is important as it represents the limiting condition in which the brightness range of an image goes from regions that are just below saturation level to regions that are barely visible.
CCDs have a high dynamic range because of their high full well capacity (FWC) and low read noise. The FWC of a CCD is a function of the physical size of the individual pixel. Larger photodiodes result in a higher FWC and, therefore, a higher dynamic range. Scientific-grade CCDs tend to employ larger photodiodes than those intended for consumer applications. This allows them to maximize dynamic range, sensitivity, and signal-to-noise ratio.
The read noise of a CCD is primarily generated by the on-chip preamplifier when it converts charge carriers into a voltage signal. Cooling the CCD can help to reduce the read noise to some extent.
Charging the Polaroid Spectra System Camera: A Step-by-Step Guide
You may want to see also
Frequently asked questions
A charge-coupled device (CCD) is a light-sensitive integrated circuit that captures images by converting photons to electrons. It is a metal oxide semiconductor chip sensor that transports electrically charged signals.
A CCD uses a photoactive region (an epitaxial layer of silicon) and a transmission region (a shift register) to capture images. An image is projected through a lens onto a capacitor array (the photoactive region), causing each capacitor to accumulate an electric charge proportional to the light intensity at that location. The array of capacitors is known as pixels, and each pixel is converted into an electrical charge whose intensity is related to the intensity of light captured by that pixel.
There are different types of CCDs, including electron-multiplying CCDs, intensified CCDs, frame-transfer CCDs, and buried-channel CCDs.
CCDs offer superior image quality and anti-noise capabilities, especially in low-light conditions. They are highly sensitive to light and efficient, with a quantum efficiency of 25-95%. They are also versatile and can be used in a wide range of applications, from digital cameras to medical equipment and satellite photography.